The Climate Challenge: Can Renewables Really do it Alone?
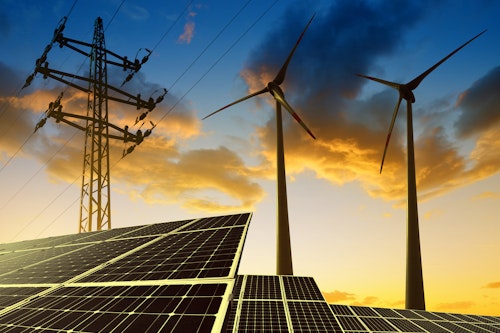
To effectively combat the looming threat of climate change, there is no doubt that we must dramatically slash carbon emissions as quickly as possible. Over the last decade, the renewable energy boom has made significant inroads towards reducing emissions, and it is evident that their share of the grid can and must go up. This is something that Third Way has advocated for since our organization’s inception. But despite these enormous gains, it is important to be clear-minded about what renewables can deliver as part of an affordable, reliable, and low-emissions electricity system. Despite tremendous technological advances in renewable generation, storage, and transmission, there are still serious challenges. A large-scale penetration of renewables into the power grid would require:
- Significant overbuilding of generation to meet demand;
- Costly grid upgrades and storage expansion;
- Storage that might not be available in time; and
- A huge build-out of transmission infrastructure.
We must continue to expand the use of renewables. But we must also recognize their limitations and put equally serious effort and political capital into bringing to scale other low-carbon technologies.
Renewables are Good, but Not Good Enough
Throughout our history, Third Way has been a vocal proponent for renewable energy. We’ve advocated strongly for greater and more effective renewable energy investment, corrected naysayers who asserted that renewables were not a viable energy option, and urged the United States to remain global leaders and innovators for renewable energy technology. And in these past few years, we have seen incredible results. The U.S. uses more renewable energy than ever before, the cost of wind and solar has plummeted, and there is significant momentum, both in public perception and private investment, for expanding renewable generation even further. Renewable energy has become a mature technology. This is a welcome and positive development, and Third Way believes that we should continue to rapidly deploy renewable energy technologies.
But as we discuss in greater detail below, the technical limitations of renewable energy, coupled with the need for rapid decarbonization of our energy system to combat climate change, requires a strong, consistent voice for other, even more radically innovative carbon reducing technologies. Wrongly, these technologies have largely been forgotten in what is too often a simplistic debate between those who are either for or against renewable energy.1 Technologies like conventional and advanced nuclear energy, carbon capture and storage, waste to energy generation, and innovative biomass cannot be left behind to pursue the vision of an energy system powered only by solar panels and wind turbines.
COP21 shows how much the international community can achieve to slash emissions. But it also presents us with the stark reality of how much farther and faster we still need to go to meet the immediate and massive climate challenge. Every credible scientific agency that has examined the impact of climate change has found that the planet is warming at an alarming rate and that we are nearing a tipping point that could lead to global catastrophe. As one of the largest per capita GHG emitters in the world and the country with the most legacy emissions, the U.S. bears an enormous responsibility for cutting our own emissions and for developing technologies that can help the rest of the world do so as well. The electric power sector, which produces 1/3 of U.S. emissions, is an obvious place to focus, as President Obama did in 2009 with the Recovery Act and in August 2015 with the Clean Power Plan. Renewable energy technologies, particularly distributed solar, utility-scale solar, and wind, are a fundamental part of cutting power sector emissions—we simply cannot do it without them. And moving to a grid that is supplied mostly by renewable energy is a very attractive vision.
But with the stakes this high for the U.S. and the planet, it is critical that we are clear-eyed about the distinction between a highly ambitious goal and one that is probably unobtainable in a timeframe that matters. And while the tremendous growth of wind and solar is excellent news—over 1,000% in the last decade—it obscures a key fact: these technologies started from a very low baseline.2 Even after all of that growth, wind and solar still combine to provide only 5% of U.S. electricity.3 Though there is great potential for further growth, even under the Clean Power Plan, renewables are still projected to hover around 15% of U.S. electricity generation in 2030.4
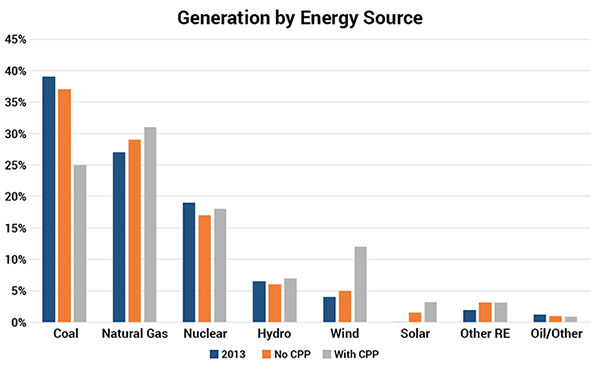
Proportion of generation for respective fuels/technologies in 2013; in 2030 under the EIA’s AEO2015 Reference case; and in 2030 under the Clean Power Plan using the Reference case as the underlying baseline. Based on EIA data.
This is not an insignificant amount, but it is by no means enough to combat the worst effects of climate change, especially when climate scientists agree that we must cut global emissions by around 60% by 2050 to stay within the 2°C target.5 A vision for the U.S. energy future that places our hopes and effort mainly on any one solution, whether it is renewables, nuclear, or natural gas-only, runs a risk of failure that we cannot afford to take. The most effective, and likely, energy system is one that is affordable, reliable, and clean. The economic and technical realities make it highly unlikely that a mostly renewables grid can achieve this, let alone do so in a timeframe that significantly mitigates climate change. Therefore, it is imperative that we develop and deploy other non-emitting technologies, because without them it will be very difficult to get the emissions reductions we need.
In this paper, we detail the major obstacles that will prevent us from scaling up to a mostly renewables U.S. grid in the foreseeable future. We explore the significant technological and economic barriers to such a grid and suggest a more feasible and less costly approach to tackle the threat of dangerous climate change.
The Many Problems of a Mostly Renewables Grid
While it might be technically possible to build a mostly renewable electricity system in the U.S.,6 the more pertinent question is whether it can be done at an affordable cost and realistic timeframe. As leading climate writer Dave Roberts has noted: “At a certain point, in a given grid system, the cost of integrating more [renewables] exceeds the benefits.”7 This is a point that the National Renewable Energy Lab (NREL) echoes in its own research.8
In fact, almost everything is subject to the law of diminishing marginal returns—one can binge-watch more Game of Thrones than is enjoyable or over-water the lawn. However, there are specific features of renewable energy that make it especially susceptible to this phenomenon. In particular, solar and wind require massive overbuilding of capacity before they can reliably supply a significant percentage of electricity on a grid system. In addition, integrating this wind and solar capacity into the grid at such high concentrations comes with its own technical challenges and costs, even when taking into account battery storage. And lastly, there are issues with land-use that make a mostly renewables grid less feasible and less affordable.
Scaling-up Requires Significant Overbuilding
All electricity sources are rated by their “capacity factor”: the ratio of the actual amount of electricity generated by, say, a wind turbine or a solar facility, versus the maximum amount of electricity that the plant theoretically could generate if it ran continuously and in ideal conditions.

Chart endnotes: 9 10
Because wind and solar are variable—the wind sometimes doesn’t blow, and nighttime and clouds limit solar—the capacity factors of these two variable renewable energy sources (VREs) are extremely low. This is particularly stark when compared to baseload technologies like zero-emission nuclear, carbon-intense coal, and natural gas, which emits half the CO2 of coal.11 This means that we would need a lot more wind or solar capacity to provide the same amount of electricity to the grid as existing baseload sources.12
Let’s examine, for example, the well-studied goal of obtaining 20% of U.S. electricity generation from wind power. (Keep in mind, that’s a pretty low target if we’re going for a grid that is around 80% VREs). Because wind is not available all the time, it might have to represent much more than half of all generation at times in order to reach the 20% annual average. But there’s another problem: wind energy tends to be most abundant at night and in the spring and fall, when demand is low.13 Reaching the 20% average therefore becomes harder still.14
This issue has already arisen in places with a high penetration of VREs. Take Denmark for example: in 2014, 39% of its electricity was generated by wind.15 But how does Denmark manage this if capacity factor is such a challenge? It is selling wind energy into a much bigger system that could absorb excess wind and account for its variability. Put simply, Denmark is not actually using all of its wind generation. It is selling wind electricity to neighboring countries when there is too much and buying electricity from them when there isn’t enough. Energy expert Roger Andrews explains:
The key to Denmark’s high level of wind penetration is its location on the Nordic Grid between its larger neighbors Norway, Sweden and Germany, who between them generated 26 times as much electricity as Denmark in 2013. This gives Denmark access to an additional 5,820 MW of interconnector capacity that it makes full use of the Nordic Grid, where other countries provide non-wind/non-renewable energy when the wind is not blowing in Denmark.16
The same thing happens in the U.S. For instance, Iowa is said to get 28.5% of its electricity from wind and that's true in terms of markets and accounting: 28.5% of the power contracts signed by Iowa utilities are with wind generators.
But there is another part to this story. Like Denmark, Iowa is part of a larger grid system known as the Midcontinent Independent System Operator (MISO) grid region, which includes all or part of 13 other states.17 The MISO grid cannot be divided into wind electrons and coal electrons. Every load (user of electricity) on the grid is, physically speaking, consuming the same mix of energy. Currently, the MISO grid gets 5.7% of its energy from wind and, thus, so does Iowa. (Technically, even MISO isn't a whole system, since it is interconnected to neighboring grids and the larger Eastern Interconnection).18
Regardless of the interconnections, the other problem for VREs is that their low capacity factor means that the challenge to the grid goes up as their penetration rate increases. Going back to Denmark, Andrews finds that increasing wind capacity to 50% of total capacity does not mean that Denmark will get 50% of its electricity from wind. This is because 20% of it would exceed demand during windy periods and have to be “curtailed” [that is, dumped].19 In fact, to reach 50% wind generation, Andrews found that Denmark would need to overbuild by nearly 60%, leading to even more wind power curtailment.20 Researchers Michael Milligan and Brendan Kirby published a study coming to the same conclusion about VREs in the U.S.21
The problem is equally acute for solar. MIT did an extensive study in 2015 of solar and concluded that at high levels of solar penetration, “it will be increasingly necessary to curtail production from solar facilities.”22 This is underscored by the case of Germany, where the oversupply of solar generation forces electricity producers to curtail extra production because prices may turn negative. If they didn’t curtail this extra solar, electricity producers would have to pay users to take the power.23 For example, German solar output surged 83 percent in the first three months of 2014, leading to 55 hours of negative prices where solar had to be curtailed.24 That’s a lot of wasted energy.
Because low capacity factor means that waste goes up with penetration, the problem of adding ever more renewables to the grid is like squeezing an increasingly dry sponge. You can always get a little bit more out of it but eventually that extra squeeze is just not worth the effort.
It is Difficult to Maintain Grid Stability with High Levels of Renewables
The flip side of wasting unneeded electricity is producing too little electricity to meet demand.25 System operators are required to meet federal reliability criteria, which usually means a reserve margin of 15% more power than the load requires at a given time. More often than not, VREs are unable to meet this threshold. A study by GE Energy Consulting and NREL found that in the U.S.’s Western Interconnect, variable renewables—at maximum peak—can provide only 55%-60% of the region’s electricity without risking the stability of the entire system.26
The problem goes back to capacity factor. In California, for example, the average wind turbine produces around 28% of its rated capacity because the wind is so variable.27 So if you have to ensure power all the time, you need some serious back-up from always-available sources. For instance, JP Morgan analyzed projected energy scenarios in renewable-heavy California and Germany, assuming 80% of electricity demand is met by renewables by 2050. Their analysis finds that in a renewables heavy grid, there will be substantial periods of unmet demand that will likely be filled by natural gas and coal generation.28
As David Roberts put it: “Since the output of VRE plants cannot be predicted with perfect accuracy in day-ahead and day-of forecasts, grid operators have to keep excess reserve running just in case.”29 The issue here is “dispatchable capacity”: the amount of power that a systems operator can order-up to meet demand. That requires continued reliance on baseload power plants (nuclear, coal, gas). An MIT study came to the same conclusion in March of 201530 and a 2014 study from the UN found the same set of problems as penetration increases.31 The threat to grid stability posed by high VRE penetration was summed-up neatly by a separate study from UC-Berkeley:
Federal, state, and provincial governments have implemented emissions targets and renewables portfolio standards that are accelerating the pace of deployment of renewables generation. Even though progress has been promising, with some grids having added tens of gigawatts of renewables, the longer term is murky; electricity grids are beginning to experience exceptional and challenging events, often caused by sudden and dramatic changes in renewables output.32
It’s easy to appreciate the dilemma. A grid operator might want to use more VREs, but their job is to keep the power on at all times. If you’re in an elevator, an operating room, or an industrial site when the power goes off, you will not want to be told to wait for the wind to start blowing.
This combination of problems relating to VREs’ capacity factor—escalating wasted electricity when renewables flood the grid and grid stability requirements when they aren’t doing enough—means that to approach an 80% renewables grid would require a massive overbuilding of both renewable capacity, storage, and load-switching. That all means that costs go up significantly for both renewables and back-up natural gas plants, which provide energy when demand peaks, as penetration rates increase.
Large-Scale Storage May Not Be Ready in Time
So if the principal problem with renewables is their variability, then perhaps energy storage can smooth out their supply curves and make them more like baseload power. This could happen at some point. But more innovation is required before storing electricity at utility-scale is close to becoming commercially viable, other than in a limited number of places using pumped storage. (Water is pumped up a hill at night using excess baseload power, and it generates hydro power when it flows back down during the day to meet peak demand.)
Can we build batteries bigger and make them feasible grid solutions anytime soon? Despite the hopeful recent headlines, that’s not at all clear. Stanford Professor Mark Jacobson did a study that called for 605,400 MWs of new storage capacity to transition to an all-renewables portfolio. That is a lot of power to store—the equivalent of the output of 600 modern nuclear reactors. U.S. grid storage as of August 2013 was a fraction of that—just 24,600 MWs, and most of that was pump storage that cannot be readily expanded. We would need to increase storage capacity nearly 25-fold, using technology that we don’t yet have.33
Both policy and technical challenges remain. The electricity sector is governed by layers of federal, regional, and state law that makes payments, contracts, and even the deployment of new technologies very complicated. As a Sandia National Laboratory report found, federal and state lawmakers and regulators are trying to incentivize more storage and allow new financing and payment structures that could make storage more economical.34 While pump storage is limited by geography, electrochemical batteries using lithium-ion, lead acid, or sodium sulfur still need to overcome various energy density, performance, charging, and cost issues.35
Even cutting-edge, much discussed battery technology still pales in comparison to the scope of the challenge.36 So while Tesla’s Powerpack is pretty extraordinary by contemporary standards—it can store the equivalent of 100 standard car batteries— scaling-up nationally would be rather daunting in the face of the costs required to get there. For example, according to a thought experiment by journalist Will Boisvert, providing power to the entire country for just 34 hours would require 160 million Powerpacks and would cost $4 trillion for the Powerpacks alone, not including installation.37 (A stack of 4 trillion dollar bills would extend far past the moon.)38
Granted, this is more than we would need—the entire grid would be unlikely to lose both wind and sun power all at once. But whatever the real number we’d have to deploy to be safe, the amount of storage required to back up a large portion of a VRE-heavy grid would be enormous.
Massive Investments in Transmission Would be Needed
On top of all this, a significant challenge is that renewable resources are not where people live. Areas of the U.S. with ample wind and solar tend to have few people—think the plains of Texas, the Montana prairie, and the Nevada desert. To get the power to the people, we would need new transmission lines. This could end up costing a tremendous amount.
The Department of Energy (DOE), NREL, and others have studied integrating wind as 20% of the electricity system. (Again, 20% wind is pretty low if we’re shooting for an 80% VRE grid).39 The DOE study estimated the cost of expanded transmission at $23 billion, while a similar study by American Electric Power (AEP) estimates such a system would require investments on the order of $60 billion.40 The even more recent Joint Coordinated System Plan estimated that integrating 20% wind into most of the eastern U.S. electricity system would cost up to $80 billion.41 Whichever study you believe, the bottom line is that transmission costs for 20% wind generation would be incredibly high.
And that’s just for wind power. We’d face similar cost challenges expanding utility-scale solar in the remote deserts of the Southwest, home to America’s best solar resources.42 Supposing that the U.S. met 70% of its electricity demand through solar by 2050, a study by the National Academies Press found that this would require an approximate 3,000 GW of added solar capacity. The study concluded that the market would not bear the costs of such an expansion and asserted that the solar plan would require a federal subsidy of $426 billion.43 That is roughly the initial cost (in 2015 dollars) of the entire interstate highway system, which is more than 40,000 miles long.44
But it’s not just cost that blocks the way. Renewables also take up a lot of room relative to their energy generation capacity. For instance, the scenario with 70% solar would cover 46,000 square miles of the Southwest U.S. (the size of Mississippi) and would need additional space for energy storage to address variability issues.45 How does land use for renewables compare with other forms of generation? Well, to produce electricity for 1,000 households per year, solar requires 8.4 acres and wind needs 6 acres, while baseload nuclear (0.69 acres), coal (0.74 acres), and natural gas (0.39 acres) are an order of magnitude lower.46 The large amount of land needed for renewables can raise a variety of local concerns about the loss of other recreational uses of land, vistas, and impact on wildlife.
Moreover, new interstate transmission becomes increasingly difficult to build when not-in-my-backyard attitudes and state politics get involved. For a power line to cross 10 states, each of those states must approve that siting, which, an NREL study found, can be incredibly complicated and time-consuming.47 The American Physical Society (the nation’s leading group of physicists) came to the same conclusion.48
Clearly, the problems of transmission and siting are not technological mysteries. We can envision many more utility-scale VRE installations and a bigger, modernized national grid. The problems here are money and time. We don’t have a lot of the first and we are most definitely running out of the second.
An All-In Climate Strategy
We recognize that even with these limitations, renewables will play a vital role in any climate change mitigation strategy. Federal programs that help fight against climate change by making renewable energy more cost competitive and accessible are important.49 And, as we illustrated earlier, battery storage technology is not currently scalable at an affordable cost, so continued public support for basic R&D is both constructive and necessary.
But the threat of climate change is far too significant to place all of our hopes for success on one set of technologies. It is imperative to expand the national discussion about climate solutions. While renewables will play a large part in reducing carbon emissions, the data we have analyzed makes a strong case that we need an “All-In” climate strategy that embraces other power generation solutions as well.
This reality contributed to Google’s decision in 2011 to end its program, known as RE>C, that sought to figure out how to get renewables cheaper than coal. As the authors of the report, Ross Koningstein and David Fork explained, “even if Google and others had led the way toward a wholesale adoption of renewable energy, that switch would not have resulted in significant reductions of carbon dioxide emissions. Trying to combat climate change exclusively with today’s renewable energy technologies simply won’t work; we need a fundamentally different approach.”50
Moreover, this debate is not confined to scaling clean energy to meet domestic electricity demand; around the world, we need to meet the needs of a population projected to grow to 9 billion people by 2070 from the 7 billion living today.51 And as emerging economies continue to develop, the average lifestyle will become increasingly energy intensive, requiring much more energy per capita than today.
To replace all of the nuclear, coal, and natural gas currently generating electricity around the world in a timeframe necessary to mitigate climate change would require building 20,000-30,000 GW of new generation by 2030 and over 50,000 by 2050.52 To put that in perspective, a nuclear plant produces one GW and costs about $10 billion; we are talking about producing enough power to match 50,000 of them. The authors of one aggressive, renewables-only plan assume costs “on the order of $100 trillion worldwide, over 20 years, not including transmission.”53 By way of comparison, the GDP of the entire world in 2013 was $74 trillion, less than ¾ of the cost of renewables-only energy.
However, significant carbon emission reductions do not have to be so costly. For example, in the case of Germany and California, JP Morgan finds that a balanced approach that embraces other low-carbon energy technologies can be just as effective while costing much less.54
Similarly, a study from the University of Adelaide found that it costs 50% more to address climate change without deploying nuclear.55 And the Intergovernmental Panel on Climate Change (IPCC) determined that it would cost up to 138% more to fight climate change without carbon capture and storage (CCS)—compared to a cost increase of just 6% if there is limited penetration of renewables.56
On a global level, it is unlikely that renewables can reach high penetrations at an acceptable cost and in a timeframe that prevents runaway climate change. Even in this country, the technical and economic challenges of a mostly renewables energy portfolio are overwhelming. When combined with the obvious political challenge of expending those kinds of resources in the fight against climate change—a fight that many leaders of one major political party still deny we should take on—it is clear that it won’t happen here either.
Conclusion
That does not mean all is lost. Renewables are improving, and their share of the grid can and must go up—hopefully reaching a substantial share of capacity. But we also must aggressively pursue other low carbon sources that can bring large quantities of baseload power to the grid in the right places and in cost-effective ways. That means adding carbon capture and sequestration to fossil plants and building new and advanced nuclear plants.57 To get there, we will have to invest in them and not place all of our money, innovation, and political efforts on the hope of U.S. and global grids powered only by the wind and sun.
Endnotes
The Economist, “Clear Thinking Needed,” November 26, 2015, Accessed December 1, 2015. Available at: http://www.economist.com/news/leaders/21679193-global-warming-cannot-be-dealt-using-todays-tools-and-mindsets-so-create-some-new?zid=313&ah=fe2aac0b11adef572d67aed9273b6e55.
United States Energy Information Administration, “Electricity Data Browser,” Accessed November 18, 2015. Available at: http://www.eia.gov/electricity/data/browser/#/topic/0?agg=2.
United States Energy Information Administration, “What is U.S. electricity generation by energy,” March 31, 2015. Accessed November 18, 2015. Available at: http://www.eia.gov/tools/faqs/faq.cfm?id=427&t=3.
Pete Danko, “EIA on Clean Power Plan: Coal Down, Renewables Up, Bills Stable,” Breaking Energy, May 26, 2015. Accessed November 18, 2015. Available at: http://breakingenergy.com/2015/05/26/eia-on-clean-power-plan-coal-down-renewables-up-bills-stable/.
Susan Hassol, “Emissions Reductions Needed to Stabilize Climate,” Presidential Climate Action Project, 2007. Accessed November 18, 2015. Available at: https://www.climatecommunication.org/wp-content/uploads/2011/08/presidentialaction.pdf.
Jacobson et al. “100% Clean and Renewable Wind, Water, and Sunlight (WWS) All Sector Energy Roadmaps for 139 Countries of the World,” Stanford University 2015. Accessed December 14, 2015. Available at: http://web.stanford.edu/group/efmh/jacobson/Articles/I/CountriesWWS.pdf.
David Roberts, “The economic limitations of wind and solar power,” Vox, June 24, 2015. Accessed November 18, 2015. Available at: http://www.vox.com/2015/6/24/8837293/economic-limitations-wind-solar.
“The limit to RE penetration is primarily economic, driven by factors that include transmission availability and operational flexibility, which is the ability of the power grid to balance supply and demand. This limit can be expressed as economic carrying capacity, or the level of variable RE generation at which that generation is no longer economically competitive or desirable to the system or society.” Quote attributed to David Roberts, “Why wind and solar power are such a challenge for energy grids,” Vox, June 19, 2015. Accessed November 18, 2015. Available at: http://www.vox.com/2015/6/19/8808545/wind-solar-grid-integration.
United States Energy Information Administration, “Capacity Factors for Utility Scale Generators Not Primarily Using Fossil Fuels January 2013-August 2015,” October 27, 2015. Accessed November 18, 2015. Available at: http://www.eia.gov/electricity/monthly/epm_table_grapher.cfm?t=epmt_6_07_b.
United States Energy Information Administration, “Capacity Factors for Utility Scale Generators Primarily Using Fossil Fuels, January 2013-August 2015,” October 27, 2015. Accessed November 18, 2015. Available at: http://www.eia.gov/electricity/monthly/epm_table_grapher.cfm?t=epmt_6_07_a.
Douglas Fischer, “Switch to Natural Gas Slashes Power Plant Pollution,” Scientific American, January 9, 2014. Accessed November 18, 2015. Available at: http://www.scientificamerican.com/article/switch-to-natural-gas-slashes-power-plant-pollution/.
It is possible that the low capacity factor of renewables could change thanks to innovation and scientific research in the sector. For instance, a new study from NREL indicates that wind capacity factors could go up to 50-65% with new turbine technology. If that proves true it would be significant, and it could help to level the playing field for wind. But NREL also found that such results would come only in limited areas that have very steady wind. And the turbines would have to be huge—about 100 feet taller than the models being erected now. That means towers the size of 46-story buildings. These hypothetical turbines would be very expensive and hard to site; just transporting them into place would be enormously difficult.
Meanwhile, solar panels could also be improved through the use of new, exotic materials like perovskite or gallium-arsenide. However, there is still a lot of research that needs to be done and the prospects of success are long term, costly, and uncertain. The U.S. and other countries must continue to invest in and support this research. But in taking on climate change, it’s important to plan based on the numbers that we are getting from renewables now.
The National Academies Press, “Electricity from Renewable Resources: Status, Prospects, and Impediments,” 2010, p. 310. Accessed November 18, 2015. Available at http://www.nap.edu/openbook.php?record_id=12619&page=310.
See also the work on this by engineer and author John Miller. John Miller, “What are the capacity factor impacts on new installed renewable generation,” Energy Collective, August 4, 2014. Access November 18, 2015. Available at: http://www.theenergycollective.com/jemillerep/450556/what-are-capacity-factor-impacts-new-installed-renewable-power-generation-capaciti.
Jesper Nørskov, “Wind turbines reached record level in 2014,” Energinet.dk, January 20, 2015, Accessed December 10, 2015. Available at: http://energinet.dk/EN/El/Nyheder/Sider/Vindmoeller-slog-rekord-i-2014.aspx.
Roger Andrews, “Wind Power, Denmark, and the Island of Denmark,” Energy Matters, February 2, 2015. Accessed November 18, 2015. Available at: http://euanmearns.com/wind-power-denmark-and-the-island-of-denmark/.
Wind supplied more than 10% of electricity consumed in Idaho, Colorado, and Oregon, according to [the wind energy trade group]. But each of these states is a member of the 160-gigawatt Western Interconnection, a synchronized grid spanning the entire western half of the continental United States plus Alberta and British Columbia, which gets only 6.6% of its electricity from wind. Indeed, double-digit penetration of VRE can only be achieved when grid subsystems are connected to larger systems. This means that areas with high levels of renewables, such as Spain, Portugal, Ireland, Denmark, and Germany, must import and export energy to neighboring grid networks in order to balance out fluctuations related to intermittency. To learn more, see the work by David Roberts, “The economic limitations of wind and solar power.”
Alex Trembath, “A Look at Wind and Solar Part 1: How Far We've Come,” The Breakthrough Institute, May 26, 2015. Accessed November 18, 2015. Available at: http://thebreakthrough.org/index.php/voices/energetics/wind-and-solar-how-far-weve-come.
Roger Andrews, “Wind Power, Denmark, and the Island of Denmark.”
To reach 50% wind penetration, Denmark would have to increase capacity from 4,500 MW to 7,650 MW. But if Denmark plans to go from its current level of 33% to 80% wind penetration, it would need to do much more than increase its capacity by a factor of around three; it would need more than five times as much as it has now. As Roger Andrews points out, the waste would be staggering, with almost 90% of every MW of added generation and over 50% of total wind generation getting curtailed.
Michael Milligan and Brendan Kirby, “Market Characteristics for Efficient Integration of Variable Generation in the Western Interconnection,” NREL, August 2010. Accessed November 18, 2015. Available at: http://www.nrel.gov/docs/fy10osti/48192.pdf.
Massachusetts Institute of Technology, “Integration of Solar Generation in Wholesale Electricity Markets,” May 5, 2015. Accessed November 18, 2015. Available at: https://mitei.mit.edu/system/files/Chapter%208_compressed.pdf.
Rachel Morison, “EU Will be Forced to Curtail Renewable Energy Generation this Summer,” Renewable Energy World, June 6, 2014. Accessed November 18, 2015. Available at: http://www.renewableenergyworld.com/articles/2014/06/eu-will-be-forced-to-curtail-renewable-energy-generation-this-summer.html.
Rachel Morison, “EU Will be Forced to Curtail Renewable Energy Generation this Summer.”
The U.S electrical grid is, actually, three completely separate networks of power lines (for the Eastern, Western, and Texas Interconnects) that connect power plants to residential and commercial customers around the country via transmission and distribution lines. The U.S. Energy Information Administration offers an excellent, detailed primer on the grid and how it operates at http://www.eia.gov/energy_in_brief/article/power_grid.cfm.
N.W. Miller, M. Shao, S. Pajic, and R. D’Aquila, “Western Wind and Solar Integration Study Phase 3 – Frequency Response and Transient Stability,” NREL, December 2014. Accessed November 18, 2015. Available at: http://www.nrel.gov/docs/fy15osti/62906.pdf/.
Wind Action, “U.S. Average Annual Capacity factors by project and State,” May 24, 2013. Accessed November 18, 2015. Available at: http://www.windaction.org/posts/37255-u-s-average-annual-capacity-factors-by-project-and-state#.VfNHNNNViko.
JP Morgan, “The Brave New World of Deep De-Carbonization of Electricity Grids,” October 19, 2015.
David Roberts, “Why wind and solar power are such a challenge for energy grids.”
Erik Delarue and Jennifer Morris, “Renewables Intermittency: Operational Limits and Implications for Long-Term Energy System Models,” MIT, March 2015. Accessed November 18, 2015. Available at: http://globalchange.mit.edu/files/document/MITJPSPGC_Rpt277.pdf.
United Nations, “UNEP: Renewables Re-energized—-Green Energy Investments Worldwide Surge 17% to $270 Billion in 2014,” April 1, 2015. Accessed November 18, 2015. Available at: http://www.un.org/climatechange/blog/2015/04/renewables-re-energized-unep-green-energy-investments-worldwide-surge-17-270-billion-2014/.
Jay Taneja, Virginia Smith, David Culler, “A Comparative Study of High Renewables Penetration Electricity Grids,” University of California, Berkely, 2013. Accessed November 18, 2015. Available at: http://www.cs.berkeley.edu/~vsmith/docs/renewables_sgc_2013.pdf.
Julian Spector, “The Environmentalist Case Against 100% Renewable Energy Plans,” Citylab, July 20, 2015. Accessed November 18, 2015. Available at: http://www.citylab.com/weather/2015/07/the-environmentalist-case-against-100-renewable-energy-plans/398906/.
United States Department of Energy, “Grid Energy Storage,” December 2013. Accessed November 18, 2015. Available at: http://www.sandia.gov/ess/docs/other/Grid_Energy_Storage_Dec_2013.pdf.
United States Department of Energy, “Grid Energy Storage.”
What about doing it as distributed storage, to accompany the distributed generation of rooftop solar? Elon Musk, the CEO of Tesla Motors, believes he can and will find a way to do provide consumer-level storage. He’s building the mother of all battery plants in Nevada, and he has promised to build a “Powerwall” that will provide uninterrupted power to homes with solar panels. It won’t be cheap. The average residential retail electricity prices in the U.S. are $0.12 per-kWh, while electricity from Tesla’s Powerwall on paired rooftop solar would cost about three times as much, $0.30 per-kWh or perhaps more. Not nearly enough consumers are ready to triple their electric bill to get reliable rooftop solar. Read more on this issue at: http://thebreakthrough.org/index.php/issues/renewables/the-grid-will-not-be-disrupted.
This estimate also does not factor in the cost of electricity needed to charge them.
Sanjoy Mahajan, “A Stack of Bills Reaching to the Moon: How to Quantify Our National Debt,” Freakonomics, July 11, 2011. Accessed November 18, 2015. Available at: http://freakonomics.com/2011/07/22/a-stack-of-bills-reaching-to-the-moon-how-to-quantify-our-national-debt/.
Michael Milligan, Erik Ela, Jeff Hein, Thomas Schneider, Gregory Brinkman, Paul Denholm, “Renewable Electricity Futures Study,” NREL, 2012. Accessed November 18, 2015. Available at: http://www.nrel.gov/docs/fy12osti/52409-4.pdf.
American Electric Power, “Interstate transmission vision for wind integration,” AEP white paper, 2007, Columbus, Ohio.
The National Academies Press, “Electricity from Renewable Resources: Status, Prospects, and Impediments,” p. 298.
Massachusetts Institute of Technology, “Concentrated Solar Power Technology,” May 5, 2015. Accessed November 18, 2015. Available at: https://mitei.mit.edu/system/files/Chapter%203_compressed.pdf.
The National Academies Press, “Electricity from Renewable Resources: Status, Prospects, and Impediments,” p. 305.
Office of Highway Policy Information, “Public Road Length - 2013 Miles By Functional System,” Federal Highway Administration, October 21, 2014. Accessed November 18, 2015. Available at: http://www.fhwa.dot.gov/policyinformation/statistics/2013/hm20.cfm.
The National Academies Press, “Electricity from Renewable Resources: Status, Prospects, and Impediments,” p. 305.
SAIC/RW Beck, “Comparison of Fuels for Power Generation,” 2013. Accessed November 18, 2015. Available at: http://www.ngsa.org/download/analysis_studies/footprint%20comparison%20april%202013.pdf.
Michael Milligan, Erik Ela, Jeff Hein, Thomas Schneider, Gregory Brinkman, Paul Denholm, “Renewable Electricity Futures Study.”
APS Panel on Public Affairs, “Integrating Renewable Electricity on the Grid,” American Physical Society, November 2011. Accessed November 18, 2015. Available at: http://www.aps.org/policy/reports/popa-reports/upload/integratingelec.pdf.
United States Department of Energy, “SunShot Initiative,” Office of Energy Efficiency and Renewable Energy. Accessed November 18, 2015. Available at: http://energy.gov/eere/sunshot/sunshot-initiative.
Ross Koningstein and David Fork, “What It Would Really Take to Reverse Climate Change,” IEEE Spectrum, November 18, 2014. Accessed November 18, 2015. Available at: http://spectrum.ieee.org/energy/renewables/what-it-would-really-take-to-reverse-climate-change.
International Institute for Applied Systems Analysis, “Population Projections.” Accessed November 18, 2015. Available at: http://www.iiasa.ac.at/web/home/research/modelsData/PopulationProjections/POP.en.html.
Peter J. Loftus, Armond M. Cohen, Jane C. S. Long, and Jesse D. Jenkins, “A critical review of global decarbonization scenarios: what do they tell us about feasibility?” Wiley Online Library, November 6, 2014. Accessed November 18, 2015. Available at: http://onlinelibrary.wiley.com/doi/10.1002/wcc.324/abstract.
Mark Z. Jacobson and Mark A. Delucchi, “A Path to Sustainable Energy by 2030,” Scientific American, November 2009. Accessed November 18, 2015. Available at: http://web.stanford.edu/group/efmh/jacobson/Articles/I/sad1109Jaco5p.indd.pdf.
JP Morgan, “The Brave New World of Deep De-Carbonization of Electricity Grids,” October 19, 2015.
Sanghyun Honga, Corey J.A. Bradshawa, Barry W. Brooka, “Global zero-carbon energy pathways using viable mixes of nuclear and renewables,” Applied Energy, April 1, 2015. Accessed November 18, 2015. Available at: http://www.sciencedirect.com/science/article/pii/S0306261915000124.
Intergovernmental Panel on Climate Change, “Climate Change 2014: Mitigation of Climate Change,” Contribution of Working Group III to the Fifth Assessment Report of the Intergovernmental Panel on Climate Change, 2014. Accessed November 18, 2015. Available at: http://mitigation2014.org/report/publication/.
Third Way, “Energy.” Accessed November 18, 2015. Available at: http://thirdway.org/issue/energy.